Condensed Matter Physics encompasses both the discovery and elucidation of fundamental processes as well as the exploration of their potential impact on technology. Our research lies at the intersection of synthesis, theory and measurement and involves the experimental study of a variety of materials, including oxides, organics, intermetallics, and semiconductor heterostructures. We have uncovered a wide range of ground states including magnetic (in frustrated and low-dimensional systems), superconducting (in heavy fermions and alkali-doped C60), and semiconducting (in topological insulators, Kondo insulators, molecular solids, Mott Hubbard systems, and 2DEGs). Our experimental techniques are thermodynamic (specific heat, magnetic, and electric susceptibility), transport (charge, heat, sound, entropy), and device prototyping. Below we describe those topics of high present interest.
Quantum Magnets
Magnetic materials have degrees of freedom and two-body interactions that are well-understood and thus useful as model systems for statistical many-body problems. They now serve as a testing ground for quantum materials, systems whose ground state wave function has no classical analogue. Several candidate quantum magnets have been found. A theme of our research is to expand on the phenomenology of quantum magnetism by developing materials in which quantum fluctuations can be tuned. A few examples are:
- Transverse Field Ising Model
- Quantum Criticality in Low-D Magnets
- New Geometrically Frustrated Magnets
- Topological Spin Glasses
Dirac Materials
The paradigm of topological insulators (TIs) not only changes the way we think of solids, but it could have far-reaching implications for electronic devices. In a manner similar to silicon’s centrality for microelectronics, the development of TIs will require simultaneous advances in fundamental understanding as well as process-guided materials selection. We are presently working on a variety of iridates, pnictides, and pyrochlores. We are also working on systems related to SmS, which have the potential to reveal topological Kondo insulating behavior. Our main goal is to develop materials that have clean gaps and thus display behavior devoid impurity conduction channels and deep traps, with an eye towards developing prototypical TI devices.
Oxide Electronics and Strong Correlations
The promise of harnessing the power of strong electronic correlations in useful devices continues to drive much activity in condensed matter. We are bridging the gap between basic phenomena and materials requirements for such devices by studying how traps and donors respond to many-body effects.
Strongly correlated systems exhibit “emergent” degrees of freedom which lead to states of matter not describable by single electron physics. Semiconductors, on the other hand, are described mainly by free electron theory determined by electronic band structure. They are useful because they can be parametrically controlled. Strongly correlated systems, by contrast, have been limited to applications that employ a ground state property, such as ferromagnetism, superconductivity, and ferroelectricity to create an external field, in essence passive devices. We are exploring strongly correlated materials for the purpose of making active devices. The materials to explore are those with the potential of a strong interaction between a correlated state and the nature of the gap, the distribution of hopping levels, as well as deep and shallow trap states.

Cobalt Niobate crystal on a mechanically-braced calorimeter prepared for measurement below 1K in the top-loading dilution refrigerator
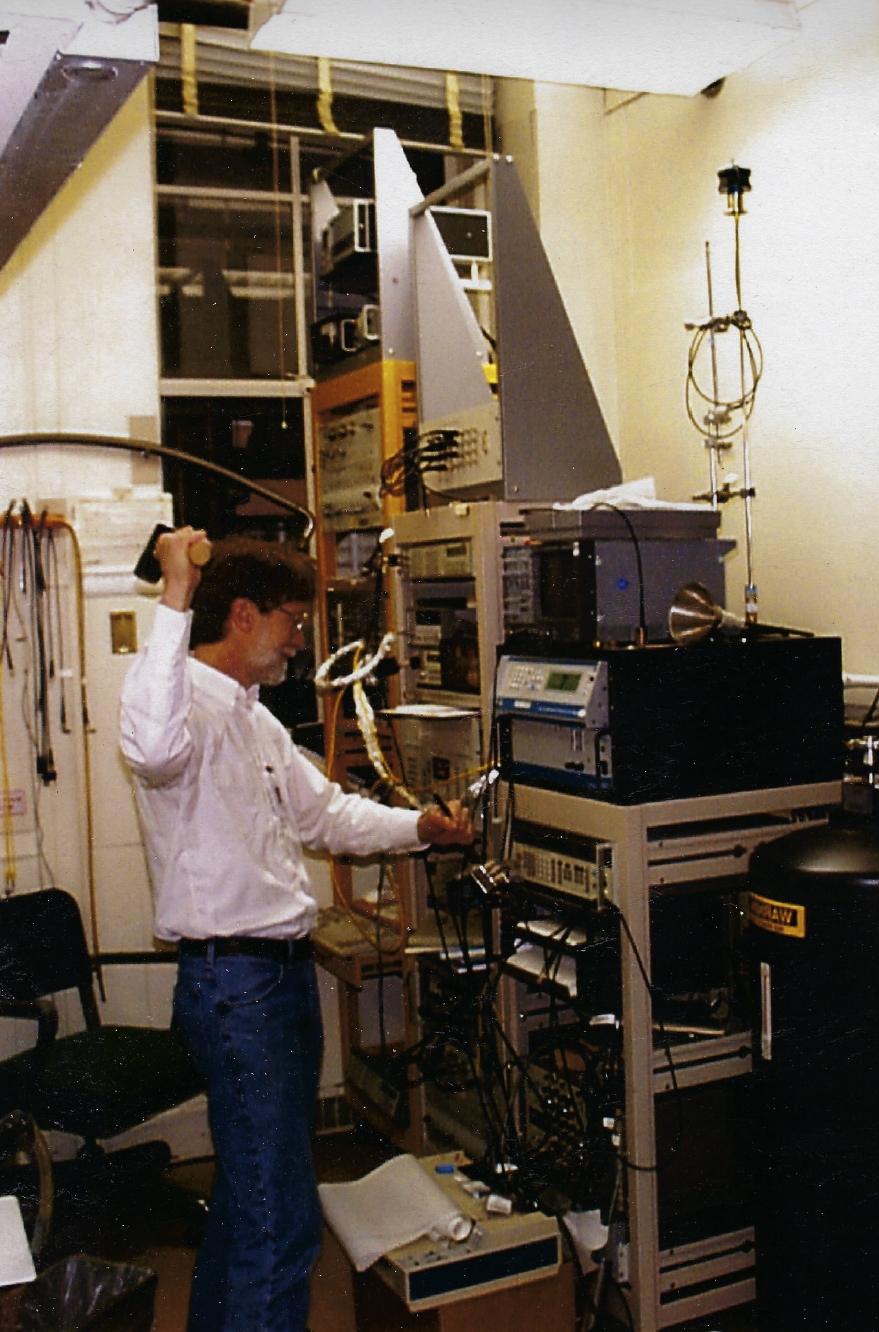
Precision measurements require great attention to detail. Here, at Bell Labs, my collaborator Allen Mills is performing a sensitive calibration.
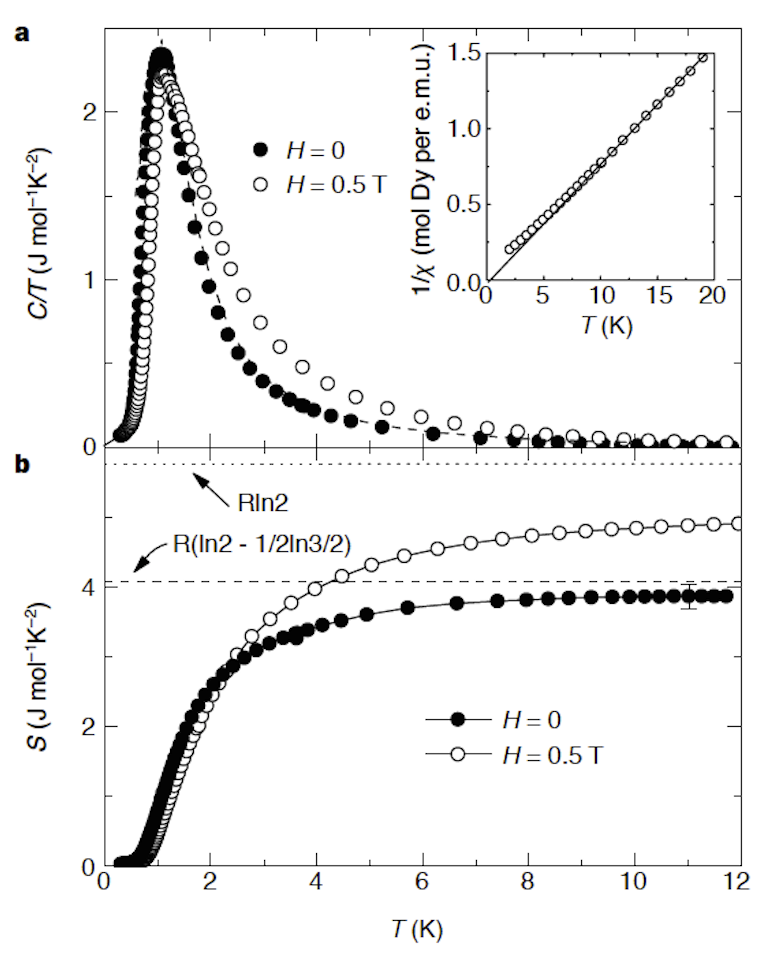